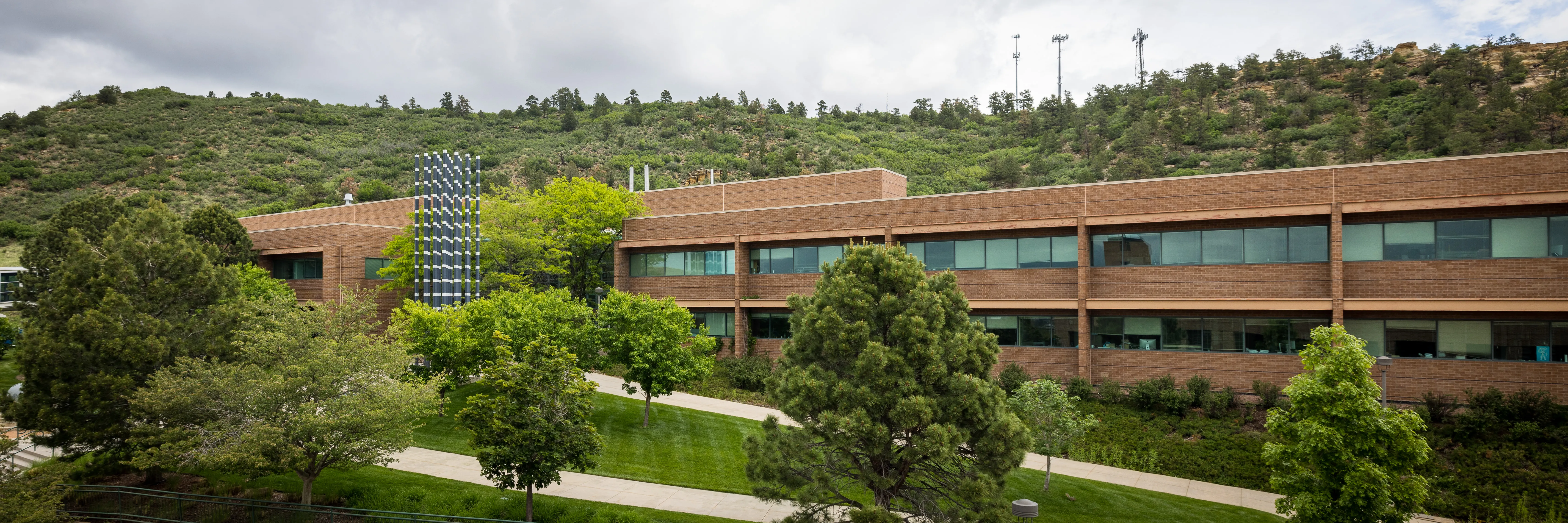
Research
Plasmonic Metal Nanoclusters for Intracellular Imaging
Metal nanoparticles and nanostructures (nanowires, nanorings, nanocubes, etc.) exhibit unique optical and electronic properties and can be used to confine light into subwavelength scale. Very often plasmonic nanoclusters exhibit photoluminescence, which can be used in biology and medicine for intracellular imaging. There are also many fundamental questions such as the role of collective coherent excitations (SPR) in the photoluminescence, the transition between molecular-like clusters and bulk-like nanoparticles and their relation to optical and electronics properties of metal nanoclusters. Our goal is to understand the fundamental physics of photoluminescence and to create more efficient and stable fluorophores for bio-imaging. We use wet and solid state chemistry (e.g. thermal reduction of silver nitrate) and different types of spectroscopy and microscopy (UV-vis, fluorescence, AFM, SEM) to characterize the nanoparticles as well as theoretical methods to predict and explain their optical response (quantum physics and classical electrodynamics).
Plasmonic Optical Biochemical Sensors
This is a somewhat similar area of research in which we also use plasmonic nanostructures. However, this project has a different goal of developing nanoscale bio-chemical optical nanosensors. We use top-down methods of nanofabrication, as opposed to bottom-up wet and solid state chemistry synthesis described above. We use Electron Beam Lithography (EBL) to fabricate nanostructured materials. The goal is to create novel optical biochemical sensors.
Creating Temperature Maps in Magnetic Resonance Imaging
MRI is a fantastic tool for creating images of organs and tissues inside the body. We are developing methods to superimpose temperature maps on top of the anatomical features. The key idea is that specially designed magnetic particles, with a magnetic transition temperature near body temperature, can give a temperature dependent contribution to the image intensity. This could be useful for MRI-guided surgeries such as thermal ablation of tumors by lasers.
Bioanalytical Instrumentation
Development of ultra sensitive optical devices to detect the presence and behavior of biological agents/cells/complexes in a fluid. We are approaching our goal to build a device which is capable to register pmole/L concentration of bioagents.
Tissue Engineering
In collaboration with NIST (Boulder), we are developing artificial tissue using composites of liquid crystals and polymers with the goal to mimic interaction of real human tissue with heat and light.
Nanoparticles Behavior Analysis
- Drug delivery - nanoparticles are an excellent tool to deliver drugs on a cellular level. We produce ultra-small ferroelectric nanoparticles, a promising method for drug carriers.
- We are studying how electrically active nanoparticles interact with cells. We have a suspicion that such active particles suppress the development of cancerous cells and may even kill them.
- We produce various kinds of active nanoparticles and study their self-assembly to model the nanoparticle behavior in a human body/blood streams/cells/etc.
Resolving bacteria in the gut with confocal fluorescence microscopy
Our gut microbiome is full of bacteria that affect our health, and these bacteria are spatially distributed throughout our gut. We study the spatial distribution of fluorescently labeled bacterial strains in the fruit fly gut by feeding them to flies, then imaging their guts under a laser confocal microscope. We resolve bacteria in the gut at single-cell resolution. Once we know where bacteria live and how they are distributed, we will investigate interactions between microbiome spatial structure and altered gut environments (e.g. in the presence of antibiotics). These findings will shed light on bacteria engraftment, the mechanism of action for microbiome-based therapies like fecal transplants and probiotics.
Super-resolution microscopy
Fluorescence optical microscopy is one of the most important tools available for the study of biological systems at the cellular level. Unfortunately, due to diffraction phenomena the resolution of fluorescence microscopes in the lateral dimension is limited to about 250 nm. As many biological structures within cells are much smaller than this, increasing resolution is of prime importance. Several methods are now available which are able to extend the resolution of optical microscopes beyond the diffraction limit, together these methods are known as super-resolution microscopy. We currently use structured illumination microscopy methods to image cells and tissues with a resolution of about 140 nm.